J. Tanprasertsuk1, T.M. Scott1, M.A.Johnson2, L.W. Poon3, P.T. Nelson4, A. Davey5, J.L. Woodard6, R. Vishwanathan7, A.K. Barbey8, K. Barger7, X.-D. Wang7, E.J. Johnson1
1. Gerald J. and Dorothy R. Friedman School of Nutrition Science and Policy, Tufts University, Boston, MA, 02111, United States of America; 2. Department of Nutrition and Health Sciences, University of Nebraska Lincoln, Lincoln, NE, 68583, United States of America; 3. Institute of Gerontology, University of Georgia, Athens, GA, 30602, United States of America; 4. Department of Pathology, Division of Neuropathology, University of Kentucky, Lexington, KY, 40536, United States of America; 5. Department of Behavioral Health and Nutrition, College of Health Sciences, University of Delaware, Newark, DE, 19716, United States of America; 6. Department of Psychology, College of Liberal Arts and Sciences, Wayne State University, Detroit, MI, 48202, United States of America; 7. Jean Mayer USDA Human Nutrition Research Center on Aging at Tufts University, Boston, MA, 02111, United States of America; 8. Beckman Institute for Advanced Science and Technology, University of Illinois at Urbana-Champaign, Urbana, IL, 61801, United States of America
Corresponding Author: Elizabeth J. Johnson, Ph.D. Research Associate Professor, Gerald J. and Dorothy R. Friedman School of Nutrition Science and Policy, Tufts University, Boston, MA, 02111, United States of America, Email: Elizabeth.Johnson@tufts.edu
J Aging Res & Lifestyle 2021;10:8-16
Published online February 12, 2021, http://dx.doi.org/10.14283/jarlife.2021.2
Abstract
Objectives: Higher vitamin E status has been associated with lower risk of Alzheimer’s disease (AD). However, evidence of the association of vitamin E concentration in neural tissue with AD pathologies is limited. Design: The cross-sectional relationship between the human brain concentrations of α- and γ-tocopherol and the severity of AD pathologies – neurofibrillary tangle (NFT) and neuritic plaque (NP) – was investigated. Setting & Participants: Brains from 43 centenarians (≥ 98 years at death) enrolled in the Phase III of the Georgia Centenarian Study were collected at autopsy. Measurements: Brain α- and γ-tocopherol concentrations (previously reported) were averaged from frontal, temporal, and occipital cortices. NP and NFT counts (previously reported) were assessed in frontal, temporal, parietal, entorhinal cortices, amygdala, hippocampus, and subiculum. NFT topological progression was assessed using Braak staging. Multiple linear regression was performed to assess the relationship between tocopherol concentrations and NP or NFT counts, with and without adjustment for covariates. Results: Brain α-tocopherol concentrations were inversely associated with NFT but not NP counts in amygdala (β = -2.67, 95% CI [-4.57, -0.79]), entorhinal cortex (β = -2.01, 95% CI [-3.72, -0.30]), hippocampus (β = -2.23, 95% CI [-3.82, -0.64]), and subiculum (β = -2.52, 95% CI [-4.42, -0.62]) where NFT present earlier in its topological progression, but not in neocortices. Subjects with Braak III-IV had lower α-tocopherol (median = 69,622 pmol/g, IQR = 54,389-72,155 pmol/g) than those with Braak I-II (median = 72,108 pmol/g, IQR = 64,056-82,430 pmol/g), but the difference was of borderline significance (p = 0.063). γ-Tocopherol concentrations were not associated with either NFT or NP counts in any brain regions assessed. Conclusions: Higher brain α-tocopherol level is specifically associated with lower NFT counts in brain structures affected in earlier Braak stages. Our findings emphasize the possible importance of α-tocopherol intervention timing in tauopathy progression and warrant future clinical trials.
Key words: Vitamin E, dementia, Alzheimer’s disease, cognitive impairment, centenarians.
Abbreviations: Aβ: amyloid-beta; AD: Alzheimer’s disease; Amy: amygdala; Ent: entorhinal cortex; FC: frontal cortex; GCS: Georgia Centenarian Study; HDL: high-density lipoprotein; Hip: hippocampus; MAP: Memory and Aging Project; NFT: neurofibrillary tangle; NP: neuritic plaque; OC: occipital cortex; PC: parietal cortex; TC: temporal cortex.
Introduction
Alzheimer’s disease (AD) is characterized by two pathological hallmarks: amyloid-β (Aβ) and neurofibrillary tangles (NFT). Aggregation of Aβ may lead to the formation of either diffuse plaques or senile plaques, also known as neuritic plaques (NP) (1, 2). NP are generally surrounded by dystrophic neurites, associated with synaptic loss, and are consistently related to cognitive deficits (3). On the other hand, NFT consists of hyperphosphorylated tau protein. Under normal conditions tau is located in the axon and facilitates the axonal transport. In tauopathy, hyperphosphorylation of tau causes NFT formation, disrupts axonal transport, and eventually leads to neuronal death (4). Compared to Aβ pathology, the spatiotemporal progression pattern of NFT has lower interindividual variability among AD patients as the disease advances (5,6). Braak and Braak have proposed a system that semi-quantitatively assesses NFT topographic progression in the brain (described in Section 2.3 NP, NFT, and Braak and Braak NFT staging) (7).
Vitamin E is a group of eight structurally-related compounds possessing potent antioxidative function. They are α-, β-, γ-, δ-tocopherols and α-, β-, γ-, δ-tocotrienols. Their dietary intakes vary across populations (70% of vitamin E as γ-tocopherol in the US, >67% as α-tocopherol in parts of Europe) (8-10). The human brain also preferentially accumulates α-tocopherol throughout lifespan (11-13), and it was shown that cortical α- and γ-tocopherol concentrations were not significantly different between AD patients and healthy controls (13,14). On the other hand, an inverse relationship between brain α-tocopherol and cognitive performance was observed in older adults who were absent of dementia, but not among those who were demented (11). Higher dietary and serum vitamin E levels are also consistently related to lower risk of developing AD later in life (15-20). These observations propose that vitamin E may play an important role in the prevention of AD before or during early but not advanced stages of the disease.
To further support this notion, oxidative stress is one of the major pathways leading to amyloid and tau pathology and both forms of vitamin E possess antioxidative properties (21 22). Administration of vitamin E reduces AD pathologies in a mouse model of AD, and improves cognition only in early, but not advanced stages of the disease (23). α-Tocopherol supplementation also delays and abolishes tau hyperphosphorylation in two mouse models of AD (21, 24). Findings from these mouse models (21, 23, 24), together with the mentioned observational studies in human subjects (15–20), suggest vitamin E’s protective role against the progress of AD pathologies. However, the biological mechanism underlying the cognitive benefits of vitamin E in human subjects remained minimally understood.
The Georgia Centenarian Study (GCS) was a population-based study recruiting centenarians from 44 counties in northeast Georgia, USA (more detail in Section 2.1 GCS decedents and brain collection) (25,26). A subset of its participants agreed to donate their brain tissues upon death, which provided a unique opportunity to investigate the possible biological mechanism of vitamin E in the human brain. The specific aim of this study was to investigate the cross-sectional relationship between the brain concentrations of the two major dietary forms of vitamin E (α- and γ-tocopherol) and the severity of AD pathologies (NFT and NP counts) in a subset of decedents who were enrolled in the GCS.
Methods
GCS decedents and brain collection
Forty-three decedents were a subset of centenarians (defined as ≥ 98 years at death) from Phase III of the GCS (2001-2007) who consented to donate their brain upon death. Decedents who did not consent to brain donation were not included in this present analysis. There were no differences in characteristics among the decedents who did or did not choose to give consent to brain donation (25). GCS study design, recruitment, and brain sample collection procedure have been described elsewhere (25,26). Briefly, the goals of the GCS were to examine the genetic contribution to longevity, association between neuropathology and cognition, functions in health and disease, and factors that contribute to adaptation and life satisfaction. Centenarians living in the 44-county area in northeast Georgia, USA were identified with two approaches. Based on census projection, the first approach identified residents of skilled nursing facilities and personal care homes as well as community-dwelling residents. The second approach was to use the date-of-birth in voter registration files. Based on these two approaches, a population-based sample of centenarians was derived and contacted (26). To ascertain that the obtained sample pool reflected pertinent characteristics of the population, samples were recruited based on five criteria (geographic, age, gender, ethnicity, and type of residence).
Mini-Mental State Examination (MMSE), an objective assessment of global cognition, was performed at enrollment and every 6 months until mortality as previously described (26). Only MMSE from the final assessment, which was within 1 year of mortality for all subjects, was reported in this manuscript. Brain samples were collected at autopsy and stored at −80°C until analysis. The study was approved by the University of Georgia Institutional Review Board on Human Subjects. Separate approval to use de-identified samples and data for the analyses was obtained from the Tufts University/Tufts Medical Center Institutional Review Board (#8900).
Tocopherol analysis
Tocopherol analysis of these brain samples has been previously described (11). Data in the present study were taken from a subset of this publication. Briefly, two predominant forms of vitamin E in human, α- and γ-tocopherols, were extracted from brain tissue homogenates sampled from the frontal cortex (FC), temporal cortex (TC), and occipital cortex (OC) using lipid extraction procedure previously established (11). The separation and quantification of tocopherols were performed by reversed-phase high-performance liquid chromatography coupled with a photodiode array detector. The limit of detection for α- and γ-tocopherols was 2.7 pmol.
NP, NFT, and Braak and Braak NFT staging
As previously explained by Neltner et al (27), NP and NFT counts were averaged from five microscopic fields that were most severely affected in each section from the following brain regions: FC (Brodmann Area 9), TC (Brodmann Areas 21-22), parietal cortex (PC, Brodmann Areas 39-40), amygdala (Amy), entorhinal cortex (Ent), hippocampus section CA1 (Hip), and subiculum (Sub). Braak and Braak staging (referred as Braak staging hereafter) was employed as a global assessment of NFT progression as previously described: Braak I-II (transentorhinal); Braak III-IV (limbic); Braak V-VI (neocortical). Adapted from Braak and Braak (7), Braak I-II is characterized by a mild to moderate development of NFT in the transentorhinal region. A modest number of NFT may be observed in the Hip section CA1. Isolated NFT may be scarcely observed in isocortical association areas including FC and TC. In Braak III-IV, both transentorhinal and Ent are severely affected by NFT. Hip section CA1 also contains numerous NFT, as well as limbic structures (Amy, putamen, nucleus accumbens, thalamus). The Sub and isocortices remain mildly affected by NFT. In Braak V-VI, transentorhinal, Ent, Hip, Sub, subcortical nuclei and isocortical cortices are severely affected by NFT. Braak staging is one of the criteria for the neuropathological diagnosis of AD from the National Institute on Aging-Reagan Institute (NIARI) (28), along with Aβ loads and Consortium to Establish a Registry for AD’s scoring of NP. NIARI criteria summarize the histological diagnosis of AD into “no”, “low”, “intermediate”, or “high” likelihood of AD.
Other variables
Other variables reported in this manuscript include age at death, sex, race, presence of diabetes and hypertension, education, type of residence at death (community dwelling or institutionalized), history of smoking and alcohol use, and APOE ε4 allele carrier. Sex, race, education, presence of APOE ε4 allele, diabetes, and hypertension were used as covariates in linear regression models (see section 2.5 Statistical analysis).
Statistical analysis
Tocopherol concentrations, NP, and NFT counts are presented as median (IQR) due to skewed distribution. Natural log transformation was applied to tocopherol concentrations, NP and NFT counts prior to all statistical tests to meet the assumption of normality. Values for other continuous variables are expressed as either mean (standard deviation). Categorical variables are expressed as n (%). Pearson’s correlation coefficients are reported to demonstrate the correlations of tocopherol concentrations among different brain regions. Multiple linear regression was used to investigate the association between tocopherol concentrations and NP or NFT counts, and to compare tocopherol concentrations among different Braak staging. β Coefficients in the regression models with and without adjustment for covariates are presented. Kruskal-Wallis test and Fisher’s exact test were used to compare subject characteristics among three Braak stage groups. Significance level was set at α = 0.05 (i.e. p < 0.05) and the adjustment for multiple comparisons across the seven brain regions were performed with the false discovery rate. All tests were performed in R version 3.5.1.
Results
Subject characteristics
Characteristics of 43 centenarians are displayed in Table 1. Mean age at death was 102.1 (2.4) years. The majority of the subjects were female and Caucasian. Body mass index was 22.0 (4.0) kg/m2 with 58% of subjects having normal body mass index (18-25 kg/m2). About half of the subjects had hypertension while only 7% had diabetes at death. Most subjects did not finish high school, while 29% had high school as their highest education and 24% had education beyond high school. Data for education were not available for one subject. MMSE from the final assessment prior to death reflected a wide range of cognitive status in this cohort (MMSE ranging from 0 to 29). Among 31 subjects whose data for smoking status and alcohol use were available, most never smoked and never consumed alcohol. Among eight subjects (19%) who were APOE ε4 carriers, one subject was ε2/ε4 while the others were ε3/ε4. Data on vitamin E supplement use were not available.
SD: standard deviation; a. BMI could not be calculated for one double amputee. b. One subject was ε2/ε4 while seven subjects were ε3/ε4; c. MMSE score 24-30: no cognitive impairment, 18-23: mild cognitive impairment, 0-17: severe cognitive impairment
Brain α- and γ-tocopherol concentrations
Concentrations of α- and γ-tocopherols in FC, TC, and OC have been previously reported (11), and are displayed in Supplemental Table 1. Concentration of α-tocopherols was greater than γ-tocopherols in all brain regions: 48.6 times in FC, 45.6 times in TC, and 48.5 times in OC. Concentrations of α- and γ-tocopherols were not significantly different between male and female decedents in any brain regions. For both α- or γ-tocopherol, concentrations were statistically correlated among three regions of the brain as shown in Supplemental Table 2 (r = 0.4-0.6 for α-tocopherol, r = 0.8-0.9 for γ-tocopherol). Therefore, the averages of α- and γ-tocopherols from three brain regions were calculated to represent their global status (Supplemental Table 1).
Relationship between tocopherol concentrations and NP and NFT counts
NP, NFT counts, and Braak stage in the GCS decedents have been qualitatively described in earlier reports (25, 27). NP and NFT counts in different brain regions are described in Table 2. Two subjects had severe hippocampal sclerosis that hindered the assessment of NP and NFT counts in Sub and Hip (29). As depicted in Figure 1, averaged α-tocopherol concentrations from three brain regions were inversely associated with NFT counts in lower brain structures including Amy (β = -2.67, p = 0.009, adjusted p = 0.030), Ent (β = -2.01, p = 0.026, adjusted p = 0.045), Hip (β = -2.23, p = 0.009, adjusted p = 0.030), and Sub (β = -2.52, p = 0.013, adjusted p = 0.030). All these associations remained significant after adjusting for covariates sex, race, education, presence of APOE ε4 allele, diabetes, and hypertension as shown in Table 3 (except in Sub where p = 0.054).
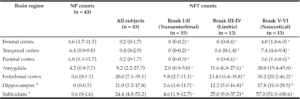
Table 2
Median (IQR) of neuritic plaque (NP) and neurofibrillary (NFT) counts. Medians that do not share a superscript are significantly different among Braak stage groups based on ANOVA post hoc tests (log transformed, p < 0.05)
IQR: interquartile range; * NP and NFT counts in hippocampus and subiculum (and therefore Braak staging) could not be assessed in two subjects due to severe hippocampal sclerosis
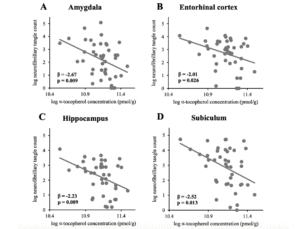
Figure 1
Associations between global α-tocopherol concentration (averaged from frontal, temporal, and occipital cortices)
and neurofibrillary tangle counts in (A) amygdala (n=43), (B) entorhinal cortex (n=43), (C) hippocampus (n=41),
and (D) subiculum (n=41)
In contrast to the abovementioned brain structures, brain global α-tocopherol concentration was not associated with NFT count in higher brain structures (FC, TC, and PC) all of which are affected by NFT in later stages of its progression (Table 3). Since NFT was absent in FC, TC, and PC in most subjects with Braak I-IV (as shown in Table 2, NFT counts in FC, TC, and PC in Braak V-VI were significantly higher than those in Braak I-IV), a sub-group analysis in subjects with Braak V-VI (n = 13) was performed. Brain global α-tocopherol concentration, as well as α-tocopherol concentration of each corresponding brain region, remained not associated with NFT count in FC, TC, and PC (p > 0.05 for all). In contrast, a sub-group analysis in subjects Braak I-IV (n = 28) demonstrated that global α-tocopherol concentration remained significantly associated with NFT counts in Amy (β = -2.06, p = 0.035), Hip (β = -2.85, p = 0.005), and Sub (β = -3.08, p = 0.004), and the association was borderline significant in Ent (β = -1.69, p = 0.086).
The association between global α-tocopherol concentration and NP count was also not observed in any brain region (Supplemental Table 3). Similarly, global γ-tocopherol concentrations were not associated with NFT nor NP count in any brain region analyzed (Supplemental Table 3), except that a borderline significance was observed with NP in Ent (β = -0.31, p = 0.053).
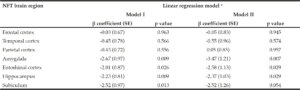
Table 3
β coefficients and p values demonstrating associations between global α-tocopherol concentrations (averaged from frontal, temporal, and occipital cortices) and neurofibrillary tangle (NFT) counts in 8 brain regions (n = 43). α-Tocopherol concentrations and NFT counts were log transformed before model fitting
SE: standard error; a. Model I: no adjustment for covariates; Model II: adjustment for sex, race, education, APOE ε4, diabetes, and hypertension.
Relationship between tocopherol concentrations and Braak NFT stages
Given the observed associations between tocopherol concentrations and NFT counts in brain regions affected in earlier Braak NFT stages, the relationship between tocopherol concentrations and Braak NFT stages (NFT topological progression) was also investigated. Among 41 subjects with Braak stage assigned, fifteen subjects (37%) were in Braak I-II (transentorhinal), thirteen (32%) were in Braak III-IV (limbic), and thirteen (32%) were in Braak V-VI (neocortical). Subject characteristics among three groups of Braak stage were not statistically different except the MMSE score and NIARI AD diagnosis (Supplemental Table 4).
As shown in Figure 2A, subjects who had Braak III-IV showed a borderline significance of lower global α-tocopherol concentrations than those of Braak I-II: 69,622 (54,389-72,155) pmol/g vs 72,108 (64,056-82,430) pmol/g (p = 0.063). This difference remained borderline significant after adjusting for covariates sex, race, education, presence of APOE ε4 allele, diabetes, and hypertension (p = 0.086). We did not observe a significant difference in α-tocopherol concentrations between subjects with Braak I-II and Braak V-VI which was 67,415 (60,877-68,922) pmol/g, either before or adjusting for covariates. As shown in Figure 2B, we also did not observe any significant differences in γ-tocopherol concentrations among three Braak stage groups either before or adjusting for covariates (Braak I-II: 1,524 (1,290-2,158) pmol/g; Braak III-IV: 1,367 (1,135-1,732) pmol/g; Braak V-VI: 1,311 (1,074-1,983) pmol/g).
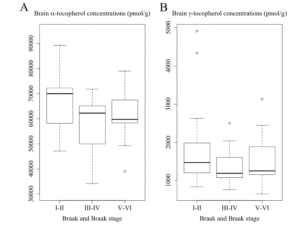
Figure 2
Concentrations of (A) α-tocopherol and (B) γ-tocopherol averaged from frontal, temporal, and occipital cortices among subjects with varying Braak staging. Subjects who had Braak III-IV showed a borderline significance of lower global α-tocopherol concentrations than those of Braak I-II (p = 0.063, ANOVA with post hoc tests on log-transformed data)
Discussion
In this cohort of centenarians, brain α-tocopherol concentration was inversely associated with NFT counts in brain structures affected during earlier but not later stages of NFT topological progression. Our findings are supported by the effect of α-tocopherol supplementation on delaying or abolishing tau hyperphosphorylation previously observed in two in mouse models of AD (21,24), although NFT count was not assessed in these studies. Early, but not late, vitamin E administration also reduced amyloid deposit in an AD mouse model (23), though NFT topological progression pattern in the human brain is more predictable and distinctive than Aβ pathology and likely to better represent the course of AD (5–7). Since increased oxidative stress (as measured by hydrogen peroxide production, lipid peroxidation, protein oxidation) precedes and may contribute to the formation of amyloid deposit and NFT (30–32), this may be a crucial period for vitamin E, being a strong lipid-soluble chain-breaking antioxidant, to manifest its action in a timely fashion. Unfortunately, no measure of oxidative stress was available in the GCS brains and we were not able to investigate the association between tocopherol status and oxidative stress. However, it has been shown that an administration of α-tocopherol significantly reduced lead-induced and brain injury-induced oxidative damage in rat brain (in vivo) (33,34), and hydrogen peroxide-induced oxidative damage in sheep brain (in vitro) (35).
Though both forms of tocopherol possess antioxidative activity as measured by different assays (36), the observed association with NFT counts in the GCS subjects was specific to α-tocopherol. The brain preferentially accumulates α-tocopherol despite γ-tocopherol accounting for 70% of dietary vitamin E in the U.S. (8), as reflected by a high brain α- to γ-tocopherol ratio in three different cohorts of older adults: γ-tocopherol was on average 1.0% in brain tissues obtained from 5 older adults (37), ranging from 1.0%-8.2% in the GCS, 2%-10% in the study by Williamson et al.(14), and 28%-38% in the Rush Memory and Aging Project (MAP) cohort (12).
To further compare our results with a similar study investigating the association between brain tocopherol concentrations and Braak NFT stages (the MAP cohort of older adults whose age at death was 88.5 (5.9) years) (12), the same analytical approach was adopted (Supplemental Table 5). We have similarly found that α-tocopherol concentrations were not linearly associated with Braak stages. However, GCS decedents with Braak III-IV, but not Braak V-VI, had a trend toward lower brain α-tocopherol concentration than those with Braak I-II (p = 0.063). While not observed in the GCS cohort, brain γ-tocopherol status was also inversely and linearly associated with Braak staging in the MAP cohort. The drastic difference of brain α- to γ-tocopherol ratio in these two cohorts should be noted, which could be due to difference in the detection method, brain regions assessed, subject characteristics, or dietary intake. Moreover, while Braak NFT staging is a useful measure of NFT topological progression, it is less informative as to the frequency of NFT in each brain region. For example, two individuals with Braak III may have very different NFT counts in the limbic system (7). Our study has its strength in the availability of both measures.
The association between NP counts in any brain region with either α- or γ-tocopherol was also not observed in the GCS decedents, as compared with the MAP study where γ-tocopherol concentrations were inversely associated with global amyloid load (12). The distinction between the two studies could be explained by the way amyloid pathology was differentially assessed (NP count in the GCS and amyloid load in the MAP) and in different brain regions. While the presence of NP is generally associated with cognitive impairment (38,39), global amyloid load is a prerequisite for AD diagnosis and catalyzes the pathological process that leads to dementia (40). An interaction between α- and γ-tocopherol concentrations on amyloid load was also observed in the MAP cohort (the association between α-tocopherol and amyloid load was dependent of γ-tocopherol status), but no interaction between α- and γ-tocopherols was observed in our study (p > 0.05 for the interaction term).
Higher dietary and serum vitamin E levels have been consistently associated with lower risk of age-related cognitive impairment and AD independently of other nutrients in numerous observational studies (15–18,41), while, though rarely observed in the U.S. population, vitamin E deficiency leads to neurological symptoms (20). On the other hand, most vitamin E interventions in subjects with or without cognitive impairment or AD have been unsuccessful in delaying or reversing the clinical symptoms (42). Findings from these trials do not contrast our findings since more than one neuropathology coexists in the brain of centenarians (27), and cognitive impairment have been associated with a wide range of pathologies in this cohort of centenarians (43). The presence of NFT in subcortical nuclei may not reflect in clinical manifestation (5, 44–47), especially in the oldest old (43,48). We have previously observed the association between cerebral and serum α-tocopherol concentrations (which likely reflect habitual intake levels) in the GCS population (49). More importantly, since the assessment of baseline vitamin E status or intakes were also not performed in these vitamin E supplement trials (42), it is difficult to accurately gauge the benefits of vitamin E supplementation. Our findings, despite being derived from a group of subjects with a wide range of cognitive status (MMSE ranging from 0 to 29), support the importance of maintaining optimal α-tocopherol status, such as through a healthy diet, as an approach to lower the risk of tauopathy-associated cognitive impairment including AD and primary age-related tauopathy, as opposed to it being a treatment after clinical symptoms have manifested. Future longitudinal studies or clinical trials in subjects free of dementia at baseline should be carried out to further substantiate this notion.
Our study has its strength in the availability of brain tocopherol concentrations in decedents with varying severity of AD pathology. Although vitamin E concentrations are only available in FC, TC, and OC, they are correlated with serum concentrations and likely to reflect the habitual intakes (49). Data in murine and rodent models also suggest a correlation of tocopherol concentrations between neocortical and other brain regions as a result of varied vitamin E intakes (50–52). For example, when a group of rats were fed a diet supplemented with α-tocopherol for 8 months, a significant increase of α-tocopherol concentration in the cerebral cortex, hippocampus, cerebellum, and striatum was similarly observed (50). Other major limitations of this study include exploratory findings in a cohort of centenarians whose characteristics were relatively homogenous (age, sex, race). Mixed neuropathologies have been observed in the cohort and the oldest old may possess certain longevity-associated traits (27, 53). Therefore, findings in this demographic group may not be directly extrapolated to lesser-aged older adults whose characteristics and pathology of dementia may be different. Multiple tests in a small cohort of subjects may also introduce false positives. However, the associations are consistently and specifically observed in brain structures where NFT affects during earlier stages of the disease, both before and after adjusting for covariates. Due to very limited numbers of patients diagnosed with primary age-related tauopathy as estimated to be 21% in centenarians (27), we were also not able to perform a sub-group analysis exclusively in these patients.
In conclusion, we observe a cross-sectional association between higher brain α-tocopherol and lower NFT counts specifically in regions affected during earlier Braak stages. Findings from the GCS cohort warrant future clinical trials that investigate vitamin E’s roles in the prevention of NFT progression and, ultimately, age-related dementia. With the recent advancement of neuroimaging technology (e.g. Positron Emission Tomography [PET] scan allowing tau deposition to be visualized in vivo) and biomarker research (e.g. plasma tau and cerebrospinal fluid tau and phosphorylated tau) (54, 55), clinical trials in which participants free or at low levels of tau pathology at baseline are tracked over time should be put forward.
Author’s contributions: The authors’ responsibility were as follows: JT, MAJ, LWP, and EJJ designed the study; MAJ, LWP, PTN, AD, and JLW contributed to the original design of the GCS and collection of neuropathological data and biological samples; RV analyzed vitamin E concentrations in all samples; JT performed statistical analysis, wrote the paper, and had primary responsibility for final content; JT, TMS, MAJ, LWP, PTN, AD, JLW, RV, AKB, KB, XDW, and EJJ interpreted the data, critically revised and approved the final manuscript.
Conflicts of interest: This work was supported by DSM Nutritional Products, NIA 1P01-AG17553, USDA 8050-51000-095-01S. All authors have no conflict of interest including any financial, personal or other relationships with people or organizations that could inappropriately influence this significant research finding. All authors have nothing to disclose.
Ethical standards: The study was approved by the University of Georgia Institutional Review Board on Human Subjects. Separate approval to use de-identified samples and data for the analyses was obtained from the Tufts University/ Tufts Medical Center Institutional Review Board (#8900).
References
1. Hebert LE, Weuve J, Scherr PA, Evans DA. Alzheimer disease in the United States (2010-2050) estimated using the 2010 census. Neurology. 2013 May 7;80(19):1778–83.
2. Vassar R, Bennett BD, Babu-Khan S, Kahn S, Mendiaz EA, Denis P, et al. β-Secretase Cleavage of Alzheimer’s Amyloid Precursor Protein by the Transmembrane Aspartic Protease BACE. Science. 1999 Oct 22;286(5440):735–41.
3. Serrano-Pozo A, Frosch MP, Masliah E, Hyman BT. Neuropathological Alterations in Alzheimer Disease. Cold Spring Harb Perspect Med. 2011 Sep;1(1).
4. Gendron TF, Petrucelli L. The role of tau in neurodegeneration. Mol Neurodegener. 2009 Mar 11;4:13.
5. Nelson PT, Alafuzoff I, Bigio EH, Bouras C, Braak H, Cairns NJ, et al. Correlation of Alzheimer Disease Neuropathologic Changes With Cognitive Status: A Review of the Literature. Journal of Neuropathology & Experimental Neurology. 2012 May;71(5):362–81.
6. Castellani RJ, Perry G. The complexities of the pathology–pathogenesis relationship in Alzheimer disease. Biochemical Pharmacology. 2014 Apr;88(4):671–6.
7. Braak H, Braak E. Neuropathological stageing of Alzheimer-related changes. Acta neuropathologica. 1991;82(4):239–259.
8. Jiang Q, Christen S, Shigenaga MK, Ames BN. Gamma-Tocopherol, the major form of vitamin E in the US diet deserves more attention. American Journal of Clinical Nutrition. 2001;74:714–22.
9. Bieri JG, Evarts RP. Tocopherols and fatty acids in American diets. The recommended allowance for vitamin E. J Am Diet Assoc. 1973 Feb;62(2):147–51.
10. Wagner K-H, Kamal-Eldin A, Elmadfa I. Gamma-tocopherol–an underestimated vitamin? Ann Nutr Metab. 2004;48(3):169–88.
11. Johnson EJ, Vishwanathan R, Johnson MA, Hausman DB, Davey A, Scott TM, et al. Relationship between Serum and Brain Carotenoids, α -Tocopherol, and Retinol Concentrations and Cognitive Performance in the Oldest Old from the Georgia Centenarian Study. Journal of Aging Research. 2013;2013:1–13.
12. Morris MC, Schneider JA, Li H, Tangney CC, Nag S, Bennett DA, et al. Brain tocopherols related to Alzheimer’s disease neuropathology in humans. Alzheimer’s & Dementia. 2015 Jan 1;11(1):32–9.
13. Metcalfe T, Bowen DM, Muller DPR. Vitamin E concentrations in human brain of patients with Alzheimer’s disease, fetuses with Down’s syndrome, centenarians, and controls. Neurochemical research. 1989;14(12):1209–1212.
14. Williamson KS, Gabbita SP, Mou S, West MS, Pye QN, Markesbery WR, et al. The Nitration Product 5-Nitro-γ-tocopherol Is Increased in the Alzheimer Brain. 2002;6(2):221-227.
15. Kontush A, Schekatolina S. Vitamin E in Neurodegenerative Disorders: Alzheimer’s Disease. Annals of the New York Academy of Sciences. 2004 Dec;1031(1):249–62.
16. Grimm M, Mett J, Hartmann T. The Impact of Vitamin E and Other Fat-Soluble Vitamins on Alzheimer´s Disease. International Journal of Molecular Sciences. 2016 Oct 26;17(11):1785.
17. Li F-J, Shen L, Ji H-F. Dietary intakes of vitamin E, vitamin C, and β-carotene and risk of Alzheimer’s disease: a meta-analysis. Journal of Alzheimer’s disease. 2012;31(2):253–258.
18. Beydoun MA, Fanelli-Kuczmarski MT, Kitner-Triolo MH, Beydoun HA, Kaufman JS, Mason MA, et al. Dietary Antioxidant Intake and Its Association With Cognitive Function in an Ethnically Diverse Sample of US Adults: Psychosomatic Medicine. 2015 Jan;77(1):68–82.
19. Perkins AJ, Hendrie HC, Callahan CM, Gao S, Unverzagt FW, Xu Y, et al. Association of antioxidants with memory in a multiethnic elderly sample using the Third National Health and Nutrition Examination Survey. American Journal of Epidemiology. 1999;150(1):37–44.
20. Brigelius-Flohé R, Traber MG. Vitamin E: function and metabolism. The FASEB Journal. 1999 Jul 1;13(10):1145–55.
21. Giraldo E, Lloret A, Fuchsberger T, Viña J. Aβ and tau toxicities in Alzheimer’s are linked via oxidative stress-induced p38 activation: Protective role of vitamin E. Redox Biology. 2014;2:873–7.
22. Tramutola A, Lanzillotta C, Perluigi M, Butterfield DA. Oxidative stress, protein modification and Alzheimer disease. Brain Research Bulletin. 2017;133:88-96.
23. Sung S, Yao Y, Uryu K, Yang H, Lee VM-Y, Trojanowski JQ, et al. Early vitamin E supplementation in young but not aged mice reduces Abeta levels and amyloid deposition in a transgenic model of Alzheimer’s disease. FASEB J. 2004 Feb;18(2):323–5.
24. Nakashima H, Ishihara T, Yokota O, Terada S, Trojanowski JQ, Lee VM-Y, et al. Effects of alpha-tocopherol on an animal model of tauopathies. Free Radic Biol Med. 2004 Jul 15;37(2):176–86.
25. Shaw K, Gearing M, Davey A, Burgess M, Poon LW, Martin P, et al. Successful Recruitment of Centenarians for Post-Mortem Brain Donation: Results from the Georgia Centenarian Study. J Biosci Med. 2012;2(1).
26. Poon LW, Jazwinski M, Green RC, Woodard JL, Martin P, Rodgers WL, et al. Methodological considerations in studying centenarians: lessons learned from the Georgia centenarian studies. Annual review of gerontology & geriatrics. 2007;27(1):231.
27. Neltner JH, Abner EL, Jicha GA, Schmitt FA, Patel E, Poon LW, et al. Brain pathologies in extreme old age. Neurobiology of Aging. 2016 Jan;37:1–11.
28. Montine TJ, Phelps CH, Beach TG, Bigio EH, Cairns NJ, Dickson DW, et al. National Institute on Aging-Alzheimer’s Association guidelines for the neuropathologic assessment of Alzheimer’s disease: a practical approach. Acta Neuropathol. 2012 Jan;123(1):1–11.
29. Nelson PT, Schmitt FA, Lin Y, Abner EL, Jicha GA, Patel E, et al. Hippocampal sclerosis in advanced age: clinical and pathological features. Brain. 2011 May 1;134(5):1506–18.
30. Praticò D, Uryu K, Leight S, Trojanoswki JQ, Lee VM-Y. Increased Lipid Peroxidation Precedes Amyloid Plaque Formation in an Animal Model of Alzheimer Amyloidosis. J Neurosci. 2001 Jun 15;21(12):4183–7.
31. Yao J, Irwin RW, Zhao L, Nilsen J, Hamilton RT, Brinton RD. Mitochondrial bioenergetic deficit precedes Alzheimer’s pathology in female mouse model of Alzheimer’s disease. Proc Natl Acad Sci USA. 2009 Aug 25;106(34):14670–5.
32. Drake J, Link CD, Butterfield DA. Oxidative stress precedes fibrillar deposition of Alzheimer’s disease amyloid beta-peptide (1-42) in a transgenic Caenorhabditis elegans model. Neurobiol Aging. 2003 Jun;24(3):415–20.
33. Patra RC, Swarup D, Dwivedi SK. Antioxidant effects of alpha tocopherol, ascorbic acid and L-methionine on lead induced oxidative stress to the liver, kidney and brain in rats. Toxicology. 2001 May 11;162(2):81–8.
34. Wu A, Ying Z. Vitamin E Protects Against Oxidative Damage and Learning Disability After Mild Traumatic Brain Injury in Rats. Neurorehabilitation and Neural Repair. 2010;24(3):290-8.
35. Sh H, H N, Aa A. Neuroprotective Role of Alpha-Tocopherol in H2O2 Induced Oxidative Stress in Neonatal Sheep’s Brain Tissue Culture. Journal of Neurology and Neurobiology. 2018;4(1).
36. Müller L, Theile K, Böhm V. In vitro antioxidant activity of tocopherols and tocotrienols and comparison of vitamin E concentration and lipophilic antioxidant capacity in human plasma. Mol Nutr Food Res. 2010 May;54(5):731–42.
37. Craft NE, Haitema TB, Garnett KM, Fitch KA, Dorey CK. Carotenoid, tocopherol, and retinol concentrations in elderly human brain. J Nutr Health Aging. 2004;8(3):156–62.
38. Serrano-Pozo A, Qian J, Muzikansky A, Monsell SE, Montine TJ, Frosch MP, et al. Thal Amyloid Stages Do Not Significantly Impact the Correlation Between Neuropathological Change and Cognition in the Alzheimer Disease Continuum. J Neuropathol Exp Neurol. 2016 Jun 1;75(6):516–26.
39. Nelson PT, Braak H, Markesbery WR. Neuropathology and cognitive impairment in Alzheimer disease: A complex but coherent relationship. Journal of Neuropathology and Experimental Neurology. 2009 Jan;68(1):1–14.
40. Spires-Jones TL, Attems J, Thal DR. Interactions of pathological proteins in neurodegenerative diseases. Acta Neuropathol. 2017 Aug 1;134(2):187–205.
41. Boccardi V, Baroni M, Mangialasche F, Mecocci P. Vitamin E family: Role in the pathogenesis and treatment of Alzheimer’s disease. Alzheimer’s & Dementia: Translational Research & Clinical Interventions. 2016 Sep 1;2(3):182–91.
42. Farina N, Llewellyn D, Isaac MGEKN, Tabet N. Vitamin E for Alzheimer’s dementia and mild cognitive impairment. Cochrane Database of Systematic Reviews. 2017;(4).
43. Tanprasertsuk J, Johnson EJ, Johnson MA, Poon LW, Nelson PT, Davey A, et al. Clinico-Neuropathological Findings in the Oldest Old from the Georgia Centenarian Study. J Alzheimers Dis. 2019;70(1):35–49.
44. Nelson PT, Jicha GA, Schmitt FA, Liu H, Davis DG, Mendiondo MS, et al. Clinicopathologic Correlations in a Large Alzheimer Disease Center Autopsy Cohort: Neuritic Plaques and Neurofibrillary Tangles “Do Count” When Staging Disease Severity. Journal of Neuropathology and Experimental Neurology. 2007 Dec;66(12):1136–46.
45. Duyckaerts C, Bennecib M, Grignon Y, Uchihara T, He Y, Piette F, et al. Modeling the relation between neurofibrillary tangles and intellectual status. Neurobiology of aging. 1997;18(3):267–273.
46. Dickson DW. Neuropathological diagnosis of Alzheimer’s disease: a perspective from longitudinal clinicopathological studies. Neurobiology of aging. 1997;18(4):S21–S26.
47. Bennett DA, Schneider JA, Wilson RS, Bienias JL, Arnold SE. Neurofibrillary tangles mediate the association of amyloid load with clinical Alzheimer disease and level of cognitive function. Arch Neurol. 2004 Mar;61(3):378–84.
48. Ganz AB, Beker N, Hulsman M, Sikkes S, Netherlands Brain Bank, Scheltens P, et al. Neuropathology and cognitive performance in self-reported cognitively healthy centenarians. Acta Neuropathologica Communications. 2018 Jul 23;6(1):64.
49. Tanprasertsuk J, Mohn ES, Matthan NR, Lichtenstein AH, Barger K, Vishwanathan R, et al. Serum Carotenoids, Tocopherols, Total n-3 Polyunsaturated Fatty Acids and n-6/n-3 Polyunsaturated Fatty Acid Ratio Reflect Brain Concentrations in a Cohort of Centenarians. J Gerontol A Biol Sci Med Sci. 2019;70(1):35-49.
50. Martin A, Prior R, Shukitt-Hale B, Cao G, Joseph JA. Effect of Fruits, Vegetables, or Vitamin E-Rich Diet on Vitamins E and C Distribution in Peripheral and Brain Tissues: Implications for Brain Function. The Journals of Gerontology Series A: Biological Sciences and Medical Sciences. 2000 Mar 1;55(3):B144–51.
51. Vatassery GT, Brin MF, Fahn S, Kayden HJ, Traber MG. Effect of High Doses of Dietary Vitamin E on the Concentrations of Vitamin E in Several Brain Regions, Plasma, Liver, and Adipose Tissue of Rats. Journal of Neurochemistry. 1988 Aug 1;51(2):621–3.
52. Vatassery GT, Angerhofer CK, Peterson FJ. Vitamin E concentrations in the brains and some selected peripheral tissues of selenium-deficient and vitamin E-deficient mice. J Neruochem. 1984;42(2):554–8.
53. Willcox DC, Willcox BJ, Poon LW. Centenarian Studies: Important Contributors to Our Understanding of the Aging Process and Longevity. Current Gerontology and Geriatrics Research. 2010;2010:1–6.
54. Lois C, Gonzalez I, Johnson KA, Price JC. PET imaging of tau protein targets: a methodology perspective. Brain Imaging Behav. 2019:13:333–44.
55. Fossati S, Cejudo JR, Debure L, Pirraglia E, Sone JY, Li Y, Chen J, Butler T, Zetterberg H, Blennow K, et al. Plasma tau complements CSF tau and p-tau in the diagnosis of Alzheimer’s disease. Alzheimers Dement (Amst). 2019;11:483–92.