K.V. Giudici1, P. de Souto Barreto1,2, S. Guyonnet1,2, C. Cantet1,2, H. Zetterberg3,4,5,6,7,8, C. Boschat9, J. Hudry9,
S. Andrieu2,10, J.A.J. Schmitt9,11,12, B. Vellas1,2, K. Blennow3,4, for the NOLAN/DSA group*
1. Gerontopole of Toulouse, Institute of Ageing, Toulouse University Hospital (CHU Toulouse), Toulouse, France; 2. CERPOP UMR1295, University of Toulouse III, Inserm, UPS, Toulouse, France; 3. Department of Psychiatry and Neurochemistry, Institute of Neuroscience and Physiology, the Sahlgrenska Academy at University of Gothenburg, Mölndal, Sweden; 4. Clinical Neurochemistry Laboratory, Sahlgrenska University Hospital, Mölndal, Sweden; 5. Department of Neurodegenerative Disease, UCL Institute of Neurology, Queen Square, London, United Kingdom; 6. UK Dementia Research Institute at UCL, London, United Kingdom; 7. Hong Kong Center for Neurodegenerative Diseases, Clear Water Bay, Hong Kong, China; 8. Wisconsin Alzheimer’s Disease Research Center, University of Wisconsin School of Medicine and Public Health, University of Wisconsin-Madison, Madison, WI, USA; 9. Brain Health Department, Nestlé Institute of Health Sciences, Nestlé Research, Société des Produits Nestlé S.A., 1000 Lausanne, Switzerland; 10. Department of Epidemiology and Public Health, Toulouse University Hospital (CHU Toulouse), Toulouse, France; 11. Singapore Institute of Food and Biotechnology Innovation (SIFBI), Agency for Science, Technology and Research (A*STAR), Singapore, Singapore; 12. Singapore Institute for Clinical Sciences (SICS), Agency for Science, Technology and Research (A*STAR), Singapore, Singapore
Corresponding Author: Kelly Virecoulon Giudici, Gérontopôle of Toulouse, Institute of Aging 37 Allée Jules Guesde 31000 Toulouse, France, E-mail: kellygiudici@gmail.com
J Aging Res & Lifestyle 2023;12:25-34
Published online June 12, 2023, http://dx.doi.org/10.14283/jarlife.2023.7
Abstract
BACKGROUN: Observational studies and some randomized controlled trials have suggested that nutritional supplementation could be a possible intervention pathway to prevent cognitive decline and Alzheimer’s disease (AD). As measuring amyloid-β and tau pathophysiology by positron emission tomography (PET) or cerebrospinal fluid (CSF) analyses may be perceived as complex, plasma versions of such biomarkers have emerged as more accessible alternatives with comparable capacity of predicting cognitive impairment.
OBJECTIVES: This study aimed to evaluate the effect of a 1-year intervention with a nutritional blend on plasma p-tau181 and glial fibrillary acidic protein (GFAP) levels in community-dwelling older adults. Effects were further assessed in exploratory analyses within sub-cohorts stratified according to p-tau status (with the third tertile considered as high: ≥15.1 pg/mL) and to apolipoprotein E (APOE) ε4 allele status.
METHODS: A total of 289 participants ≥70 years (56.4% female, mean age 78.1 years, SD=4.7) of the randomized, double-blind, multicenter, placebo-controlled Nolan trial had their plasma p-tau181 assessed, and daily took either a nutritional blend (composed of thiamin, riboflavin, niacin, pantothenic acid, pyridoxine, biotin, folic acid, cobalamin, vitamin E, vitamin C, vitamin D, choline, selenium, citrulline, eicosapentaenoic acid – EPA, and docosahexaenoic acid – DHA) or placebo for 1 year.
RESULTS: After 1-year, both groups presented a significant increase in plasma p-tau181 and GFAP values, with no effect of the intervention (p-tau181 between-group difference: 0.27pg/mL, 95%CI: -0.95, 1.48; p=0.665; GFAP between-group difference: -3.28 pg/mL, 95%CI: -17.25, 10.69; p=0.644). P-tau- and APOE ε4-stratified analyses provided similar findings.
CONCLUSION: In community-dwelling older adults, we observed an increase in plasma p-tau181 and GFAP levels that was not different between the supplementation groups after one year.
Key words: p-tau, cognitive decline, Alzheimer, nutrition, clinical trials.
Introduction
In a worldwide context of increasing life expectancy and Alzheimer’s disease (AD) burden, identifying lifestyle factors that promote brain health is of high relevancy, and may help preventing or postponing cognitive decline, and consequently increasing quality of life. Observational studies associating nutritional factors to better cognitive function have suggested that nutritional supplementation could be a possible intervention pathway to prevent cognitive decline and Alzheimer’s disease (AD) (1-7). While ambiguous results have been found in trials supplementing single nutrients (8), other studies evaluating the combination of several nutrients have suggested that this might be a better strategy (9-13). The Nolan Study was then designed to test this novel approach, through offering a nutritional blend (NB) developed to serve as a source of multiple nutrients to community dwelling older adults, and previously tested on cats (12) and dogs (13). In spite of not being able to affect cognitive function, as measured by clinical tests (except for a positive effect on the Cognitive Function Instrument – CFI study partner score), the nutritional blend positively improved two biomarkers that are believed to underlie an attenuation in cognitive decline during aging (14-16): plasma homocysteine (Hcy) levels decreased and erythrocyte omega-3 index increased after the 1-year intervention (17).
AD is a serious neurological disorder characterized by the gradual accumulation of amyloid-β (Aβ) plaques in the brain, which promotes neuronal toxicity, tau phosphorylation and aggregation, and neuronal death (18, 19). As measuring Aβ and tau pathology in the brain by positron emission tomography (PET) is expensive and have limited availability, and cerebrospinal fluid (CSF) collection may be regarded as complex to perform, plasma versions of such biomarkers have emerged as alternatives with comparable capacity of predicting cognitive impairment (20-23). Plasma tau phosphorylated at threonine 181 (p-tau181) has been identified as a predictor of cognitive decline (24) and of tau accumulation in the brain (25), with high performance to identify AD, while levels are normal in other dementias (26, 27). High p-tau181 levels were also found in the very early stages of the disease, even preclinically (28), and correlated tightly with other p-tau variants, such as p-tau231 and p-tau217 (29). Glial fibrillary acidic protein (GFAP) is a cytoskeletal protein commonly expressed in astroglia, which has also been associated with cognitive decline, amyloidosis and AD (30-33). Thus, it is of great interest to investigate whether the supplementation with the nutritional blend would be able to promote changes on plasma p-tau181 and on GFAP levels. Moreover, considering the important impact of the apolipoprotein E (APOE) ε4 allele on AD risk (34), subgroup analyses according to APOE ε4 status would also bring additional elements to the field.
This study aimed to evaluate the effect of a 1-year intervention with a nutritional blend composed of several nutrients (thiamin, riboflavin, niacin, pantothenic acid, pyridoxine, biotin, folic acid, cobalamin, vitamin E, vitamin C, vitamin D, choline, selenium, citrulline, eicosapentaenoic acid – EPA, and docosahexaenoic acid – DHA) on plasma p-tau181 and GFAP levels among community-dwelling older adults. Effects were further assessed in exploratory analyses within sub-cohorts stratified according to p-tau181 status (low vs. high) and to APOE ε4 status (carriers vs. non-carriers).
Materials and Methods
Study design and population
The Nolan Study is a double-blind, multicenter, randomized, placebo-controlled trial (RCT) conducted in 18 centers in France. Participants were community-dwelling older adults, recruited from December 2016 to January 2018. Follow-up ended in February 2019. Major inclusion criteria comprised: being ≥70 years old; self-reporting subjective memory complaints; and having a study partner to participate as a source of information. Major exclusion criteria were: taking vitamin-B supplements in the past three months; taking ω-3 PUFA supplements containing >200 mg of DHA/day over six months before inclusion; basic activities of daily living (ADL) score <4; Mini-Mental State Examination (MMSE) score <24; confirmed diagnosis of dementia. Detailed inclusion and exclusion criteria has been described elsewhere (17). Treatment with anticoagulants or platelet aggregation inhibitors were accepted, but carefully monitored, considering that ω-3 PUFA can affect platelet function.
Out of the 362 participants who joined the Nolan study, 57 dropped-out during follow-up (n=26 intervention, n=31 control; mean drop-out rate = 15.7%), totaling 305 participants at the end of the trial (n=154 intervention, n=151 control). From those, 289 had their plasma p-tau181 and GFAP measured, and were then included in this study. However, six aberrant values for GFAP at the 12-month visit were considered as outliers, and were not included in the analyses. A detailed description of the sample formation is provided in Figure 1.
Ethical disclosure
The Nolan Study was registered at www.clinicaltrials.gov (protocol NCT03080675) and approved by the Advisory Committee for Protection of Persons South West and Overseas II (CPP SOOM II) and by the French Agency for the Safety of Medicines and Health Products (ANSM). All participants signed an informed consent.
Randomization and masking
For the original main analysis of the Nolan Study, participants were randomly assigned (1:1) to either the intervention group (receiving the supplementation with the NB) or the control group (receiving placebo). Details are given in Supplementary materials.
Intervention
Participants allocated in the intervention group were instructed to take a nutritional blend daily, for one year. The blend was characterized by two soft gel capsules (with 775mg filling each) and by one powdered sachet of ≈15g, to be mixed in 120mL of cold water. In the meanwhile, participants in the control group took placebo equivalent volumes. Total composition of a daily dose of the NB consisted of 50mg of thiamin, 15 mg of riboflavin, 25mg of niacin, 23mg of pantothenic acid, 18mg of pyridoxine, 0.15mg of biotin, 0.4mg of folic acid, 0.5mg of cobalamin, 82.6mg of vitamin E, 500mg of vitamin C, 15μg of VD, 85mg of choline, 80μg of selenium, 3g of citrulline, 700mg of EPA and 770mg of DHA. Additional information about capsules and powered drink composition is presented in Supplementary materials.
In addition to a total of six in-person visits over the follow-up (screening, practice, baseline, 1 month, 6 months and 12 months), phone calls were set up between visits at 3-month intervals to follow-up product compliance, adverse events and concomitant medication intake. Although dietary restrictions have not been made, participants were instructed not to take any additional supplements containing B-vitamins, DHA and/or EPA.
Outcomes: plasma p-tau181 and GFAP
Plasma samples were collected at baseline, 6 months, and 12 months of follow-up, but p-tau181 and GFAP were assessed only at baseline and 12 months. Plasma p-tau181 concentration was measured in the Clinical Neurochemistry Laboratory, University of Gothenburg (Mölndal, Sweden), using an in-house Simoa method based on the AT270 (specific for the threonine-181 phosphorylation site) and Tau12 (N-terminal epitope 6-18 on human tau protein), as described previously in detail (26). Plasma GFAP concentration was measured using the commercially available GFAP Discovery assay (Quanterix, Billerica, MA). All measurements were performed on an HD-X Analyzer (Quanterix, Billerica, MA) in one round of experiments with baseline and follow-up samples from the same individual side-by-side on the assay plates by board-certified laboratory technicians who were blinded to clinical information. Intra-assay coefficients of variation were below 10%.
To define subgroups of participants with normal (lower) and high p-tau status, and in the absence of a consensual range in literature to determine it, tertiles were used, with participants in the first and second tertiles considered as low (<15.1 pg/mL) and those in the third tertile considered as high (≥15.1 pg/mL).
APOE ε4 allele status and other study variables
The effect of intervention on plasma p-tau181 was also investigated in exploratory analysis according to the APOE ε4 allele status (carrier vs. non-carrier). In addition, differences in plasma p-tau181 at baseline were investigated according to sex (female, male), age ranges (70 to 74 years, 75 to 79 years, 80 to 84 years, ≥85 years), education (≤7 years, 8 to 9 years, ≥10 years), body mass index (BMI) (<21 kg/m2, 21 to 24.9 kg/m2, 25 to 29.9 kg/m2, ≥30 kg/m2), Clinical Dementia Rating (CDR) score (0, 0.5) and amyloid status assessed by positron emission tomography (PET) (positive, negative).
Statistical analysis
Means (SD – standard deviation) and frequencies (percentages) were used to characterize the studied population. Baseline characteristics of participants according to allocation groups were compared by Chi-Square test or Student’s t-test. Differences in plasma p-tau181 according to participants’ characteristics were tested using ANOVA or Student’s T-test. Efficacy analyses were done using data of all randomly assigned participants with plasma p-tau181 and GFAP measurements who completed the 12-month follow-up. Linear regression models adjusted on baseline data were performed in order to determine the effect of intervention, compared to placebo, on plasma p-tau181 and GFAP levels, including all available data (baseline and 12 months). The following fixed effects were included in the model: baseline value, intervention group, time (continuous variable), and interaction between group and time. Similarly, exploratory analysis according to plasma p-tau181 status and to APOE ε4 allele status was performed. The Statistical Analysis Software (SAS) version 9.4 (Cary, NC, USA) was used, with statistical significance set as 5%.
Results
Characterization of the sample
Table 1 presents baseline characteristics of participants according to randomized groups. The study sample of 289 Nolan participants had mean age 78.1 years (SD=4.7) and was composed of 56.4% female (n=163). Plasma p-tau181 did not differ between treatment groups at baseline (intervention: 14.1 pg/mL, SD=6.0 vs. control: 13.3 pg/mL, SD=5.6, p=0.262), but baseline plasma GFAP was higher in the intervention group (intervention: 194.4 pg/mL, SD=87.6 vs. control: 171.0 pg/mL, SD=67.7, p=0.013).
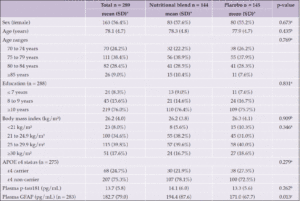
Table 1. Baseline characteristics of participants of the Nolan Study with plasma p-tau181 assessment
APOE, apolipoprotein E gene; GFAP, glial fibrillary acidic protein; p-tau181, phosphorylated tau at threonine 181; SD, standard deviation; *Except where indicated other. a Chi-Square test; b Equal variance two sample t-test, c Unequal variance two sample t-test.
Differences in plasma p-tau181 were observed according to age range, sex, APOE ε4 status, CDR score and the presence of amyloid plaques assessed by PET scan, with higher plasma p-tau181 values observed among subjects ≥85 years old (p=0.002), male (p=0.023), APOE ε4 carriers (p=0.002), with CDR score 0.5 (p=0.001) and amyloid-positive (p=0.046). No differences were observed according to education and BMI. For plasma GFAP, differences were observed according to age range, sex, BMI, and CDR score, with higher GFAP observed among subjects ≥85 years old (p=0.001), female (p=0.005), with BMI <21 kg/m² (p<0.0001) and with CDR score 0.5 (p=0.023). No differences were observed according to education, APOE ε4 status and amyloid status (determined by PET) (Table 2). Participants of the present study did not differ in baseline characteristics from those participants of Nolan who were not included due to the absence of the investigated biomarkers (Table 3).
APOE, apolipoprotein E gene; CDR, Clinical Dementia Rating; GFAP, glial fibrillary acidic protein; PET, positron emission tomography; p-tau181, phosphorylated tau at threonine 181; SD, standard deviation; n=289, a Equal variance two sample t-test;.b ANOVA F-test; c Unequal variance two sample t-test.
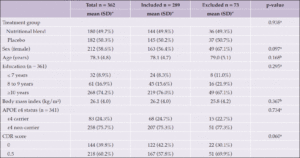
Table 3. Comparison of baseline characteristics between participants of the Nolan Study with plasma p-tau181 assessment (included in the present study) and participants not included
APOE, apolipoprotein E gene; CDR, Clinical Dementia Rating; SD, standard deviation; *Except where indicated other. a Chi-Square test; b Equal variance two sample t-test.
Effect of intervention on plasma p-tau181 and GFAP
After 1 year, plasma p-tau181 significantly increased in both groups, with no differences between participants who received the nutritional blend or those who received placebo (between-group difference: 0.27 pg/mL, 95%CI: -0.95, 1.48; p=0.665). Similar findings were observed for plasma GFAP, increasing in both groups over time and with no effect of intervention (between-group difference: -3.28 pg/mL, 95%CI: -17.25, 10.69; p=0.644) (Table 4).

Table 4. Mixed-effect linear regression analysis for change from baseline in plasma p-tau181 and GFAP according to intervention groups among non-demented, community-dwelling older adults
GFAP, glial fibrillary acidic protein; p-tau181, phosphorylated tau at threonine 181; 1Estimated with the mean at baseline.
Exploratory analysis according to APOE ε4 allele status
The allele APOE ε4 was identified among 24.7% of the sample (n=68), and did not differ between groups (intervention: 21.9% vs. control: 27.5%; p=0.279) (Table 1). Additional analyses according to APOE ε4 status mostly provided similar findings to the observed among the total studied population, with no differences in plasma p-tau181 and GFAP changes between groups (difference in between-group differences over time for APOE ε4 carriers vs. non-carriers: p-tau181: 2.28 pg/mL, 95%CI: -0.58, 5.13; p=0.118; GFAP: 3.16 pg/mL, 95%CI: -28.24, 34.56; p=0.843) (Table 5).

Table 5. Mixed-effect linear regression analysis for change from baseline in plasma p-tau181 according to intervention groups and APOE ε4 status among non-demented, community-dwelling older adults
GFAP, glial fibrillary acidic protein; p-tau181, phosphorylated tau at threonine 181; 1Estimated with the mean at baseline; 2Defined as presenting at least one ε4 allele.
Exploratory analysis according to plasma p-tau181 status
Exploratory analyses according to plasma p-tau181 status also found no effect of intervention on plasma p-tau181 and GFAP over 1-year, independently of p-tau status as baseline (difference in between-group differences over time for high vs. low: p-tau181: 0.81pg/mL, 95%CI: -1.74, 3.36; p=0.532; GFAP: -12.49 pg/mL, 95%CI: -41.69, 16.72; p=0.401) (Table 6). Interestingly, significant 1-year increase in plasma p-tau181 was only observed among participants with high p-tau181 at baseline (Table 6).

Table 6. Mixed-effect linear regression analysis for change from baseline in plasma p-tau181 according to intervention groups and plasma p-tau181 status among non-demented, community-dwelling older adults
GFAP, glial fibrillary acidic protein; p-tau181, phosphorylated tau at threonine 181; 1Estimated with the mean at baseline; 2Defined as the two first tertiles (<15.1 pg/mL); 3Defined as the third tertile (≥15.1 pg/mL).
Discussion
Our study found no effect of a 1-year supplementation with a nutritional blend on plasma p-tau181 and GFAP levels among community-dwelling older adults, with both plasma biomarkers significantly increasing in both groups at the end of follow-up. Analyses according to APOE ε4 status provided similar findings. Analyses stratifying subjects according to their p-tau181 status at baseline revealed that only those with high p-tau presented significant increase in plasma p-tau181 after 1-year.
It is well understood that nutrition is a key factor affecting aging (35-37) and the development of neurodegenerative diseases (38-40). However, the influence of dietary factors on tau accumulation has not been largely explored so far. There are studies with animals pointing towards the beneficial role of nutrient supplementation. For example, in a study with amyloid precursor protein (APP) and presenilin 1 (PS1) double-transgenic mice (a well-established AD mouse model), vitamin D supplementation for 20 weeks decreased the expression levels of cortical APP, tau and p-tau (41). In addition, a 9-month DHA supplementation in 3xTg-AD mice was shown to reduce intraneuronal tau accumulation (42). It should be noted, however, that these studies were performed in AD animal models, a different approach from our investigation (focused on prevention and on the potential action of supplementation prior to disease onset, which also might demand higher exposure time compared to treatment scenarios). In humans, RCT have provided mixed findings. A RCT offering a 4-week high saturated fatty acid/glycemic index diet or a low SFA/GI diet for cognitively normal adults and adults with mild cognitive impairment (MCI) found that diets significantly modified CSF amino acid levels, and reported that such changes in amino acids were associated with changes in CSF tau and p-tau181 (43). On the other hand, a RCT investigating the effects of a 6-month supplementation with 2.3g/day of PUFAs on CSF biomarkers among AD patients found no effect of treatment on p-tau or t-tau (44). Other RCTs found no benefits of supplementation of single or combined nutrients or bioactive compounds (omega-3 PUFA (45); vitamin E, vitamin C, α-lipoic acid and coenzyme Q (46); copper (47); resveratrol (48)) on CSF t-tau and/or p-tau.
Multiple potential biological mechanisms are believed to be involved on this relationship between nutrients and tau accumulation (49). Vitamin E has been reported to prevent Aβ-induced tau phosphorylation both in vitro and in APP/PS1 transgenic mice, by inhibiting p38MAPK phosphorylation (50). Intraperitoneal injections containing trans retinoic acid (a vitamin A metabolite) were shown to reduce tau hyperphosphorylation by decreasing the activity of cyclin-dependent kinase 5 (CDK5 – a kinase involved in the abnormal phosphorylation of tau), in a study with APP/PS1 mice (51). Moreover, it has been shown that vitamin D reduced Aβ (25-35)-induced tau hyperphosphorylation through interplaying with glial cell line-derived neurotrophic factor (GDNF) signaling in SH-SY5Y cells (52). In accordance, Wu et al. (53) have recently shown that the activation of vitamin D receptor (VDR) reduced tau phosphorylation by inhibiting the GSK3β phosphorylation (Tyr216) in APP/PS1 mice. All such findings highlight the importance of further exploring how nutrition may contribute to fight tauopathies.
The evaluation of plasma GFAP in the present study brings additional elements to our investigation. GFAP is recognized as a marker of astrocyte reactivity (also known as astrocytosis or astrogliosis), a pathological process that is commonly found surrounding Aβ plaques in the brain of patients with AD (54). These activated glial cells are believed to be a defensive mechanism to fight amyloidosis, but when persistent, are pro-inflammatory and contribute to the worsening of AD progression (54). Therefore, the increasing of GFAP over time is associated with unhealthy prognosis (30–33). Our 1-year supplementation with the nutritional blend, however, did not mitigate the increase in plasma GFAP over time. Investigations on the effects of nutrient supplementation on GFAP are still scarce in literature, but there is evidence linking vitamin D (55) and EPA (56) supplementation with inhibition of glial activation in rodents. Future studies on the topic are hence encouraged.
Known as a strong genetic risk factor for the development of AD (34), the presence of the allele ε4 in the APOE gene importantly affects several metabolic pathways, including impaired cerebral glucose metabolism, altered microglia function (promoting increased tau pathology), decreased cerebral blood flow and impaired ability of astrocytes to synthesize and secrete cholesterol (57). For this reason, people carrying the APOE ε4 allele are believed to benefit from specific nutritional recommendations, as higher DHA, vitamin D and B-vitamins intake, no alcohol consumption (57), lower saturated fat intake and the adherence to the Mediterranean dietary pattern (58). However, additional research offering high-level evidence is still needed before the consensual establishment of adapted dietary recommendations for heterozygous and homozygous APOE ε4 carriers. Despite all the metabolic particularities triggered by the APOE ε4 allele, we found no differences from main findings when analyses were separately performed according to APOE ε4 status. Neither did Yassine et al. (59) when evaluating DHA supplementation on CSF tau and p-tau levels among individuals with probable AD.
Although the supplementation tested in the present trial was able to improve omega-3 index and to reduce plasma Hcy levels (17), its 1-year duration may have been insufficient to affect plasma p-tau181 and GFAP, as also the given doses of each nutrient or compound. The fact that participants of the study were generally healthy individuals may also have exacerbated this relatively short follow-up. To our knowledge, the present study is the first to test the effect of a multi-nutrient supplementation on plasma p-tau181 levels, a biomarker that reflects amyloid and tau protein deposition in the brain (25, 60, 61), and is advantageous compared to CSF and brain measurements due to its lower costs and complexity. The randomized, controlled design of the study stands as one of its strengths. On the other hand, the fact that other p-tau variants (as p-tau217 and p-tau231) were not assessed in our study may be noted as a limitation. As other limitations, we must consider the relatively short duration of intervention, and the missing data for some of the study variables, especially amyloid status assessed by PET scan. The choice of the threshold of p-tau181 status for the exploratory analysis (based on tertiles, once there are no validated established cutoffs so far) should be also noted. Moreover, most participants of the Nolan Study presented high educational level, so caution is needed to avoid generalizing the results to other populations.
Conclusions
In this study, we observed a 1-year increase in plasma p-tau181 and GFAP levels among community-dwelling older adults that was not different between subjects receiving supplementation with a nutritional blend composed of several vitamins and minerals or those receiving placebo. Considering the novelty of the hypothesis tested in the present study, additional RCT with longer follow-ups and including other p-tau and astrocytosis biomarkers are needed, and may contribute to a better understanding if (and how) nutritional supplementation may be able to protect brain health and cognitive function through impacting tau accumulation, amyloidosis and glial activation. Additional research would also shed light on the identification of subgroups to whom specific nutritional supplementation may be more effective. Together with other advances in the field targeting lifestyle approaches, this would enable not only setting nutritional strategies for optimizing brain health and preventing or slowing the development of neurodegenerative diseases, but also to reduce the need of drugs and expensive treatments.
* Nolan/DSA Study group: Nolan Study Group: Principal investigator: Bruno Vellas (Toulouse); Co-principal investigator: Sophie Guyonnet; Study coordinator: Lauréane Brigitte; CRA: Céline Cluzan; Methodology and statistical analysis: Sandrine Andrieu, Christelle Cantet. Investigators in coordinating centres: Pierre-Jean Ousset, Catherine Faisant, Françoise Lala, Julien Delrieu, Nathalie Sastre, Yves Rolland, Sandrine Sourdet, Marie Champarnaud, Philippe Girard; Psychologists: Emeline Combrouze, Audrey Casaux (Toulouse); Investigators in associated centre: Sébastien Laborie, Carine Chiffre, Marc Alonso (Albi); Jean-François Dartigues, Sophie Auriacombe, Isabelle Bourdel-Marchasson, Muriel Rainfray, Nathalie Salles, Claire Roubaud Baudron, Sandrine Harston, (Bordeaux); Véronique Kostek, Frédérique Olivier, Albine Louchet, Inga Couffignal (Cahors) ; Marie-Noëlle-Cuffi, Amandine Lefort, Sophie Dardenne, Magali Fleury, Corine Costes (Castres); Yannick Bejot, Olivier Rouaud, Cécile Chazalon, Benoit Delpont, Agnès Jacquin-Piques, Catia Khoumri (Dijon); Lawrence Bories, Evelyne Campistron, Marie-Laure Pader (Foix); Anne Hustache, Dominique Penchenat (Gourdon); Aurianne Ravaud, Jean Bernard Chauderon (Labastide-Murat); Françoise Desclaux (Lavaur); Florence Pasquier, Thibaud Lebouvier, Vincent Deramecourt, Pascaline Cassagnaud, Marie-Anne Mackowiak, Adeline Rollin, Stéphanie Bombois, Catherine Adnet-Bonte (Lille); Thierry Dantoine (Limoges); Marc Bonnefoy, Thomas Gilbert (Lyon Sud Pierre Bénite); Pierre Krolak Salmon, Jing Xie, Denis Federico, Isabelle Rouch, Christophe Magnier, Marie Hélène Coste, Zaza Makaroff, Keren Danaila Kenny David, Julien Vernaudon (Lyon Villeurbanne); Hélène Mollion, Isabelle Roullet-Solignac, Maïté Formaglio (Lyon Est); Mathieu Ceccaldi, Claude Gueriot, Radka Gantchev Kletchkova (Marseille); Aurélie Roustan, Kristel Scheirlinckx – Sudres, Carole Cervera (Montauban); Laurin Brignol (Moissac); Audrey Gabelle, Karim Bennys, Aude Metzger, Cecilia Marelli, Claire Hourregue (Montpellier); Michael Li Yung Tong, Cynthia Laurent, Rosanne Ufkes (Muret); Michel Dutech, Patrick Gervais, Vincent Courrière (Nailloux) ; Gilles Berrut, Laure De Decker, Sophie Pichierri, Pascal Chevalet, Typhaine Riaudel, Marie-Hélène Fix, Fanny Pesle, Catherine Couturier (Nantes); Philippe Robert, Renaud David, Aurélie Mouton, Georges Niewiadomski (Nice); Olivier Guerin, Guillaume Sacco, Cyprien Arlaud, Cyrielle Rambaud, Marine Sanchez, Emilie Ferrer (Nice Hôpital Cimiez); Olivier Hanon, Marie Laure Seux, Clémence Boully, Emmanuelle Duron, Lena Joffredo, Anne Chahwakilian, Jean-Sébastien Vidal, Galdric Orvoen, Edouard Chaussade, Florian Labourée, Laure Caillard, Hermine Lenoir, Sophie Chauvelier, Yasmina Boudali (Paris) ; Jacques Hugon, Claire Paquet, Emmanuel Cognat, Julien Dumurgier (Paris Lariboisière); Bruno Dubois, Katia Andrade, Stéphane Epelbaum (Paris La Pitié Salpétrière); Frédéric Blanc (Strasbourg); Yves Gasnier, Kai Ostendorf, Jean-Pierre Salles, Samira Misbah El Idrissi, Isabelle Zagar, Thérèse Uguen, Stéphanie Sallaberry-Benech, Vincent Dodier, KhaledKhales (Tarbes); Stéphane Oustric, Marie-Eve Rouge Bugat, Marc Viguier, Emile Escourrou, Laetitia Gimenez, Michel Combier (Toulouse); Nestlé project team: Jeroen Schmitt, Corina Boschat, Julie Hudry, Dominik Grathwohl, Cecilia Fumero, Emilie Darcillon, Julia Mauger, Ludivine Feraille-Naze, John Corthesy (Lausanne). MRI & PET scans group: Pierre Payoux, Anne-Sophie Salabert (Toulouse ; Stéphane Lehéricy, Marie Chupin, Jean-François Mangin, Ali Bouhayia (Paris); Michèle Allard (Bordeaux); Frédéric Ricolfi (Dijon); Dominique Dubois (Foix); Marie Paule Bonceour Martel (Limoges); François Cotton (Lyon); Alain Bonafé (Montpellier); Stéphane Chanalet (Nice); Françoise Hugon (Tarbes); Fabrice Bonneville, Christophe Cognard, François Chollet (Toulouse); Biological sample collection: Bertrand Perret, Bénédicte Razat (Toulouse); Safety management : Pascale Olivier-Abbal (Toulouse, France). DSA Group: Sandrine Andrieu, Christelle Cantet, Nicola Coley.
Funding statement: The global sponsor of the Nolan Study is Société des Produits Nestlé SA, Nestlé Research, Lausanne, Switzerland. The sponsor for France is the University Hospital Center of Toulouse. The study sponsor was involved in study center set-up and monitoring, preparation and distribution of BPB and placebo, data collection and monitoring working closely with the investigators. Initial training sessions and yearly investigator’s meeting were organized by both the University Hospital Center of Toulouse and Nestlé. The data sharing activity was supported by the Association Monegasque pour la Recherche sur la maladie d’Alzheimer (AMPA) and the INSERM-University of Toulouse III UMR 1027 research unit.
Conflicts of interest: CB, JH and JAJS work at the Société des Produits Nestlé SA, Nestlé Research, Lausanne, Switzerland. KB has served as a consultant, at advisory boards, or at data monitoring committees for Abcam, Axon, BioArctic, Biogen, JOMDD/Shimadzu, Julius Clinical, Lilly, MagQu, Novartis, Ono Pharma, Pharmatrophix, Prothena, Roche Diagnostics, and Siemens Healthineers, and is a co-founder of Brain Biomarker Solutions in Gothenburg AB (BBS), which is a part of the GU Ventures Incubator Program, outside the work presented in this paper. HZ has served at scientific advisory boards and/or as a consultant for Abbvie, Acumen, Alector, Alzinova, ALZPath, Annexon, Apellis, Artery Therapeutics, AZTherapies, CogRx, Denali, Eisai, Nervgen, Novo Nordisk, Optoceutics, Passage Bio, Pinteon Therapeutics, Prothena, Red Abbey Labs, reMYND, Roche, Samumed, Siemens Healthineers, Triplet Therapeutics, and Wave, has given lectures in symposia sponsored by Cellectricon, Fujirebio, Alzecure, Biogen, and Roche, and is a co-founder of Brain Biomarker Solutions in Gothenburg AB (BBS), which is a part of the GU Ventures Incubator Program (outside submitted work). BV is an investigator in clinical trials sponsored by Nestle, Biogen, Lilly, Roche, Eisai , Pfizer, Pierre Fabre Pharmaceuticals and the Toulouse University Hospital. He has served as SAB Scientifc Advisory Board Meeting member for Biogen , Alzheon, Green Valey, Norvo Nordisk, Longeveron, Rejuvenate Biomed Clinical Pfizer, Eisai France, but received no personal compensation. He has served as consultant and/or SAB member for Roche, Lilly, Eisai, TauX, Cerecin with personal compensation. The curent study was funded by Nestlé to the Toulouse University Hospital without personal compensation. Other authors declare no conflict of interest.
Authors’ contributions: SA, BV and JAJS conceived the Nolan Study and designed research; CC analyzed data; KVG wrote the paper; KVG and CC had primary responsibility for final content. KB and HZ developed the p-tau181 Simoa immunoassay and supervised analyses in the present study. RB managed data of plasma p-tau181. KVG, SG, CC, PSB, RB, KB, HZ, CB, JH, SA, BV and JAJS interpreted the data and revised the draft critically for intellectual content. All authors read and approved the final manuscript.
Clinical Trial Registry number: NCT03080675 registered at www.clinicaltrials.gov
References
1. da Costa KA, Gaffney CE, Fischer LM, Zeisel SH. Choline deficiency in mice and humans is associated with increased plasma homocysteine concentration after a methionine load. Am J Clin Nutr. 2005 Feb;81(2):440–4. doi: 10.1093/ajcn.81.2.440.
2. Clarke R, Bennett D, Parish S, Lewington S, Skeaff M, Eussen SJPM, et al. Effects of homocysteine lowering with B vitamins on cognitive aging: meta-analysis of 11 trials with cognitive data on 22,000 individuals. Am J Clin Nutr. 2014 Aug;100(2):657–66. doi: 10.3945/ajcn.113.076349.
3. Mocchegiani E, Costarelli L, Giacconi R, Malavolta M, Basso A, Piacenza F, et al. Vitamin E-gene interactions in aging and inflammatory age-related diseases: implications for treatment. A systematic review. Ageing Res Rev. 2014 Mar;14:81–101. doi: 10.1016/j.arr.2014.01.001.
4. Dyall SC. Long-chain omega-3 fatty acids and the brain: a review of the independent and shared effects of EPA, DPA and DHA. Front Aging Neurosci. 2015;7:52. doi: 10.3389/fnagi.2015.00052.
5. Solovyev ND. Importance of selenium and selenoprotein for brain function: From antioxidant protection to neuronal signalling. J Inorg Biochem. 2015 Dec;153:1–12. doi: 10.1016/j.jinorgbio.2015.09.003.
6. Moretti M, Fraga DB, Rodrigues ALS. Preventive and therapeutic potential of ascorbic acid in neurodegenerative diseases. CNS Neurosci Ther. 2017 Dec;23(12):921–9. doi: 10.1111/cns.12767.
7. Kalra A, Teixeira AL, Diniz BS. Association of Vitamin D Levels with Incident All-Cause Dementia in Longitudinal Observational Studies: A Systematic Review and Meta-analysis. J Prev Alzheimers Dis. 2020;7(1):14–20. doi: 10.14283/jpad.2019.44.
8. Vauzour D, Camprubi-Robles M, Miquel-Kergoat S, Andres-Lacueva C, Bánáti D, Barberger-Gateau P, et al. Nutrition for the ageing brain: Towards evidence for an optimal diet. Ageing Res Rev. 2017 May;35:222–40. doi: 10.1016/j.arr.2016.09.010.
9. Smith AD, Smith SM, de Jager CA, Whitbread P, Johnston C, Agacinski G, et al. Homocysteine-lowering by B vitamins slows the rate of accelerated brain atrophy in mild cognitive impairment: a randomized controlled trial. PloS One. 2010 Sep 8;5(9):e12244. doi: 10.1371/journal.pone.0012244.
10. Oulhaj A, Jernerén F, Refsum H, Smith AD, de Jager CA. Omega-3 Fatty Acid Status Enhances the Prevention of Cognitive Decline by B Vitamins in Mild Cognitive Impairment. J Alzheimers Dis JAD. 2016;50(2):547–57. doi: 10.3233/JAD-150777.
11. Li M, Li W, Gao Y, Chen Y, Bai D, Weng J, et al. Effect of folic acid combined with docosahexaenoic acid intervention on mild cognitive impairment in elderly: a randomized double-blind, placebo-controlled trial. Eur J Nutr. 2021 2021 Jun;60(4):1795-1808. doi: 10.1007/s00394-020-02373-3.
12. Pan Y, Araujo JA, Burrows J, de Rivera C, Gore A, Bhatnagar S, et al. Cognitive enhancement in middle-aged and old cats with dietary supplementation with a nutrient blend containing fish oil, B vitamins, antioxidants and arginine. Br J Nutr. 2013 Jul 14;110(1):40–9. doi: 10.1017/S0007114512004771.
13. Pan Y, Kennedy AD, Jönsson TJ, Milgram NW. Cognitive enhancement in old dogs from dietary supplementation with a nutrient blend containing arginine, antioxidants, B vitamins and fish oil. Br J Nutr. 2018;119(3):349–58. doi: 10.1017/S0007114517003464.
14. Smith AD, Refsum H. Homocysteine, B Vitamins, and Cognitive Impairment. Annu Rev Nutr. 2016 17;36:211–39. doi: 10.1146/annurev-nutr-071715-050947.
15. Setién-Suero E, Suárez-Pinilla M, Suárez-Pinilla P, Crespo-Facorro B, Ayesa-Arriola R. Homocysteine and cognition: A systematic review of 111 studies. Neurosci Biobehav Rev. 2016 Oct;69:280–98. doi: 10.1016/j.neubiorev.2016.08.014.
16. Hooper C, De Souto Barreto P, Pahor M, Weiner M, Vellas B. The Relationship of Omega 3 Polyunsaturated Fatty Acids in Red Blood Cell Membranes with Cognitive Function and Brain Structure: A Review Focussed on Alzheimer’s Disease. J Prev Alzheimers Dis. 2018;5(1):78–84. doi: 10.14283/jpad.2017.19.
17. Giudici KV, Guyonnet S, Cantet C, de Souto Barreto P, Weiner MW, Tosun D, Boschat C, Hudry J, Andrieu S, Vellas B, Schmitt JAJ; Nolan/DSA group. A 1-year randomized controlled trial of a nutritional blend to improve nutritional biomarkers and prevent cognitive decline among community-dwelling older adults: the NOLAN Study. Alzheimers Dement (N Y). 2022;8(1):e12314. doi: 10.1002/trc2.12314.
18. Jack CR, Bennett DA, Blennow K, Carrillo MC, Dunn B, Haeberlein SB, et al. NIA-AA Research Framework: Toward a biological definition of Alzheimer’s disease. Alzheimers Dement J Alzheimers Assoc. 2018;14(4):535–62. doi: 10.1016/j.jalz.2018.02.018.
19. Selkoe Dj, Hardy J. The amyloid hypothesis of Alzheimer’s disease at 25 years. EMBO Mol Med. 2016;8(6):595-608. doi: 10.15252/emmm.201606210.
20. Ovod V, Ramsey KN, Mawuenyega KG, Bollinger JG, Hicks T, Schneider T, et al. Amyloid β concentrations and stable isotope labeling kinetics of human plasma specific to central nervous system amyloidosis. Alzheimers Dement J Alzheimers Assoc. 2017 Aug;13(8):841–9. doi: 10.1016/j.jalz.2017.06.2266.
21. Nakamura A, Kaneko N, Villemagne VL, Kato T, Doecke J, Doré V, et al. High performance plasma amyloid-β biomarkers for Alzheimer’s disease. Nature. 2018 08;554(7691):249–54. doi: 10.1038/nature25456.
22. Bateman RJ, Blennow K, Doody R, Hendrix S, Lovestone S, Salloway S, et al. Plasma Biomarkers of AD Emerging as Essential Tools for Drug Development: An EU/US CTAD Task Force Report. J Prev Alzheimers Dis. 2019;6(3):169–73. doi: 10.14283/jpad.2019.21.
23. Wang X, Sun Y, Li T, Cai Y, Han Y. Amyloid-β as a Blood Biomarker for Alzheimer’s Disease: A Review of Recent Literature. J Alzheimers Dis JAD. 2020;73(3):819–32. doi: 10.3233/JAD-190714.
24. Smirnov DS, Ashton NJ, Blennow K, Zetterberg H, Simrén J, Lantero-Rodriguez J, et al. Plasma biomarkers for Alzheimer’s Disease in relation to neuropathology and cognitive change. Acta Neuropathol (Berl). 2022 Apr;143(4):487–503. doi: 10.1007/s00401-022-02408-5.
25. Moscoso A, Karikari Tk, Grothe Mj, Ashton Nj, Lantero-Rodriguez J, Snellman A, et al. CSF biomarkers and plasma p-tau181 as predictors of longitudinal tau accumulation: Implications for clinical trial design. Alzheimers Dement. 2022 Dec;18(12):2614-2626. doi: 10.1002/alz.12570.
26. Karikari TK, Pascoal TA, Ashton NJ, Janelidze S, Benedet AL, Rodriguez JL, et al. Blood phosphorylated tau 181 as a biomarker for Alzheimer’s disease: a diagnostic performance and prediction modelling study using data from four prospective cohorts. Lancet Neurol. 2020;19(5):422–33. doi: 10.1016/S1474-4422(20)30071-5.
27. Benussi A, Karikari TK, Ashton N, Gazzina S, Premi E, Benussi L, et al. Diagnostic and prognostic value of serum NfL and p-Tau181 in frontotemporal lobar degeneration. J Neurol Neurosurg Psychiatry. 2020;91(9):960–7. doi: 10.1136/jnnp-2020-323487.
28. O’Connor A, Karikari TK, Poole T, Ashton NJ, Lantero Rodriguez J, Khatun A, et al. Plasma phospho-tau181 in presymptomatic and symptomatic familial Alzheimer’s disease: a longitudinal cohort study. Mol Psychiatry. 2021;26(10):5967–76. doi: 10.1038/s41380-020-0838-x.
29. Suárez-Calvet M, Karikari TK, Ashton NJ, Lantero Rodríguez J, Milà-Alomà M, Gispert JD, et al. Novel tau biomarkers phosphorylated at T181, T217 or T231 rise in the initial stages of the preclinical Alzheimer’s continuum when only subtle changes in Aβ pathology are detected. EMBO Mol Med. 2020 07;12(12):e12921. doi: 10.15252/emmm.202012921.
30. Rajan KB, Aggarwal NT, McAninch EA, Weuve J, Barnes LL, Wilson RS, et al. Remote Blood Biomarkers of Longitudinal Cognitive Outcomes in a Population Study. Ann Neurol. 2020 Dec;88(6):1065–76. doi: 10.1002/ana.25874.
31. Benedet AL, Milà-Alomà M, Vrillon A, Ashton NJ, Pascoal TA, Lussier F, et al. Differences Between Plasma and Cerebrospinal Fluid Glial Fibrillary Acidic Protein Levels Across the Alzheimer Disease Continuum. JAMA Neurol. 2021 01;78(12):1471–83. doi: 10.1001/jamaneurol.2021.3671.
32. Cicognola C, Janelidze S, Hertze J, Zetterberg H, Blennow K, Mattsson-Carlgren N, et al. Plasma glial fibrillary acidic protein detects Alzheimer pathology and predicts future conversion to Alzheimer dementia in patients with mild cognitive impairment. Alzheimers Res Ther. 2021 Mar 27;13(1):68. doi: 10.1186/s13195-021-00804-9.
33. Pereira JB, Janelidze S, Smith R, Mattsson-Carlgren N, Palmqvist S, Teunissen CE, et al. Plasma GFAP is an early marker of amyloid-β but not tau pathology in Alzheimer’s disease. Brain J Neurol. 2021 16;144(11):3505–16. doi: 10.1093/brain/awab223.
34. Farrer La, Cupples La, Haines Jl, Hyman B, Kukull Wa, Mayeux R, et al. Effects of age, sex, and ethnicity on the association between apolipoprotein E genotype and Alzheimer disease. A meta-analysis. APOE and Alzheimer Disease Meta Analysis Consortium. JAMA. 1997 Oct;278(16):1349-56. doi:10.1001/jama.1997.03550160069041
35. Gurău F, Baldoni S, Prattichizzo F, Espinosa E, Amenta F, Procopio AD, et al. Anti-senescence compounds: A potential nutraceutical approach to healthy aging. Ageing Res Rev. 2018 Sep;46:14–31. doi: 10.1016/j.arr.2018.05.001.
36. Shannon OM, Ashor AW, Scialo F, Saretzki G, Martin-Ruiz C, Lara J, et al. Mediterranean diet and the hallmarks of ageing. Eur J Clin Nutr. 2021 Aug;75(8):1176–92. doi: 10.1038/s41430-020-00841-x.
37. Virecoulon Giudici K. Editorial: Nutrition and the Hallmarks of Aging. J Nutr Health Aging. 2021;25(9):1039–41. doi: 10.1007/s12603-021-1686-3.
38. Gutierrez L, Folch A, Rojas M, Cantero JL, Atienza M, Folch J, et al. Effects of Nutrition on Cognitive Function in Adults with or without Cognitive Impairment: A Systematic Review of Randomized Controlled Clinical Trials. Nutrients. 2021 Oct 22;13(11). doi: 10.3390/nu13113728.
39. Ding H, Reiss AB, Pinkhasov A, Kasselman LJ. Plants, Plants, and More Plants: Plant-Derived Nutrients and Their Protective Roles in Cognitive Function, Alzheimer’s Disease, and Other Dementias. Med Kaunas Lith. 2022 Jul 30;58(8). doi: 10.3390/medicina58081025.
40. Gates EJ, Bernath AK, Klegeris A. Modifying the diet and gut microbiota to prevent and manage neurodegenerative diseases. Rev Neurosci. 2022 Mar 21; 33(7):767-787. doi: 10.1515/revneuro-2021-0146.
41. Lin J, Niu Z, Xue Y, Gao J, Zhang M, Li M, et al. Chronic vitamin D3 supplementation alleviates cognition impairment via inhibition of oxidative stress regulated by PI3K/AKT/Nrf2 in APP/PS1 transgenic mice. Neurosci Lett. 2022 13;783:136725. doi: 10.1016/j.neulet.2022.136725.
42. Green Kn, Martinez-Coria H, Khashwji H, Hall Eb, Yurko-Mauro Ka, Ellis L, et al. Dietary docosahexaenoic acid and docosapentaenoic acid ameliorate amyloid-beta and tau pathology via a mechanism involving presenilin 1 levels. J Neurosci. 2007 Apr 18;27(16):4385-95. doi: 10.1523/JNEUROSCI.0055-07.2007.
43. Russin KJ, Nair KS, Montine TJ, Baker LD, Craft S. Diet Effects on Cerebrospinal Fluid Amino Acids Levels in Adults with Normal Cognition and Mild Cognitive Impairment. J Alzheimers Dis JAD. 2021;84(2):843–53. doi: 10.3233/JAD-210471.
44. Tofiq A, Zetterberg H, Blennow K, Basun H, Cederholm T, Eriksdotter M, et al. Effects of Peroral Omega-3 Fatty Acid Supplementation on Cerebrospinal Fluid Biomarkers in Patients with Alzheimer’s Disease: A Randomized Controlled Trial-The OmegAD Study. J Alzheimers Dis JAD. 2021;83(3):1291–301. doi: 10.3233/JAD-210007.
45. Freund-Levi Y, Hjorth E, Lindberg C, Cederholm T, Faxen-Irving G, Vedin I, et al. Effects of omega-3 fatty acids on inflammatory markers in cerebrospinal fluid and plasma in Alzheimer’s disease: the OmegAD study. Dement Geriatr Cogn Disord. 2009;27(5):481–90. doi: 10.1159/000218081.
46. Galasko DR, Peskind E, Clark CM, Quinn JF, Ringman JM, Jicha GA, et al. Antioxidants for Alzheimer disease: a randomized clinical trial with cerebrospinal fluid biomarker measures. Arch Neurol. 2012 Jul;69(7):836–41. doi: 10.1001/archneurol.2012.85.
47. Kessler H, Pajonk Fg, Bach D, Schneider-Axmann T, Falkai P, Herrmann W, et al. Effect of copper intake on CSF parameters in patients with mild Alzheimer’s disease: a pilot phase 2 clinical trial. J Neural Transm (Vienna). 2008 Dec;115(12):1651-9. doi: 10.1007/s00702-008-0136-2.
48. Turner Rs, Thomas Rg, Craft S, van Dyck Ch, Mintzer J, Reynolds Ba, et al. A randomized, double-blind, placebo-controlled trial of resveratrol for Alzheimer disease. Neurology. 2015 Oct 20;85:1383–91. doi: 10.1212/WNL.0000000000002035.
49. Fernández-Sanz P, Ruiz-Gabarre D, García-Escudero V. Modulating Effect of Diet on Alzheimer’s Disease. Dis Basel Switz. 2019 Jan 26;7(1):12. doi: 10.3390/diseases7010012.
50. Giraldo E, Lloret A, Fuchsberger T, Viña J. Aβ and tau toxicities in Alzheimer’s are linked via oxidative stress-induced p38 activation: protective role of vitamin E. Redox Biol. 2014;2:873–7. doi: 10.1016/j.redox.2014.03.002.
51. Ding Y, Qiao A, Wang Z, Goodwin JS, Lee ES, Block ML, et al. Retinoic Acid Attenuates β-Amyloid Deposition and Rescues Memory Deficits in an Alzheimer’s Disease Transgenic Mouse Model. J Neurosci. 2008 Nov 5;28(45):11622–34. doi: 10.1523/JNEUROSCI.3153-08.2008.
52. Lin CI, Chang YC, Kao NJ, Lee WJ, Cross TW, Lin SH. 1,25(OH)2D3 Alleviates Aβ(25-35)-Induced Tau Hyperphosphorylation, Excessive Reactive Oxygen Species, and Apoptosis Through Interplay with Glial Cell Line-Derived Neurotrophic Factor Signaling in SH-SY5Y Cells. Int J Mol Sci. 2020 Jun 13;21(12):4215. doi: 10.3390/ijms21124215.
53. Wu TY, Zhao LX, Zhang YH, Fan YG. Activation of vitamin D receptor inhibits Tau phosphorylation is associated with reduction of iron accumulation in APP/PS1 transgenic mice. Neurochem Int. 2022;153:105260. doi: 10.1016/j.neuint.2021.105260.
54. Osborn LM, Kamphuis W, Wadman WJ, Hol EM. Astrogliosis: An integral player in the pathogenesis of Alzheimer’s disease. Prog Neurobiol. 2016;144:121–41. doi: 10.1016/j.pneurobio.2016.01.001.
55. Vieira Adc, Medeiros Eb, Zabot Gc, de Souza Pereira N, do Nascimento Nb, Lidio Av, et al. Neuroprotective effects of combined therapy with memantine, donepezil, and vitamin D in ovariectomized female mice subjected to dementia model. Prog Neuropsychopharmacol Biol Psychiatry. 2023 Mar 2;122:110653. doi: 10.1016/j.pnpbp.2022.110653.
56. Dong Y, Xu M, Kalueff AV, Song C. Dietary eicosapentaenoic acid normalizes hippocampal omega-3 and 6 polyunsaturated fatty acid profile, attenuates glial activation and regulates BDNF function in a rodent model of neuroinflammation induced by central interleukin-1β administration. Eur J Nutr. 2018 Aug;57(5):1781–91. doi: 10.1007/s00394-017-1462-7.
57. Norwitz NG, Saif N, Ariza IE, Isaacson RS. Precision Nutrition for Alzheimer’s Prevention in ApoE4 Carriers. Nutrients. 2021 Apr 19;13(4). doi: 10.3390/nu13041362.
58. Fote GM, Geller NR, Reyes-Ortiz AM, Thompson LM, Steffan JS, Grill JD. A Scoping Review of Dietary Factors Conferring Risk or Protection for Cognitive Decline in APOE ε4 Carriers. J Nutr Health Aging. 2021;25(10):1167–78. doi: 10.1007/s12603-021-1705-4.
59. Yassine HN, Rawat V, Mack WJ, Quinn JF, Yurko-Mauro K, Bailey-Hall E, et al. The effect of APOE genotype on the delivery of DHA to cerebrospinal fluid in Alzheimer’s disease. Alzheimers Res Ther. 2016 30;8:25. doi: 10.1186/s13195-016-0194-x.
60. Janelidze S, Mattsson N, Palmqvist S, Smith R, Beach TG, Serrano GE, et al. Plasma P-tau181 in Alzheimer’s disease: relationship to other biomarkers, differential diagnosis, neuropathology and longitudinal progression to Alzheimer’s dementia. Nat Med. 2020;26(3):379–86. doi: 10.1038/s41591-020-0755-1.
61. Mielke MM, Dage JL, Frank RD, Algeciras-Schimnich A, Knopman DS, Lowe VJ, et al. Performance of plasma phosphorylated tau 181 and 217 in the community. Nat Med. 2022;28(7):1398–405. doi: 10.1038/s41591-022-01822-2.
© Serdi 2023